In the construction industry, concrete is highly valued. It is, after water, the most consumed material on the planet, but its main drawback is its brittleness, which is due to its poor resistance to crack formation, low tensile strength, and low strain capacities. Many efforts have been made to improve the performance of cement-based materials by modifying the properties of cement composites using admixtures, supplementary cementitious materials, and fibers.
Fiber-reinforced concrete has a higher tensile strength and toughness. Fibers also replace large cracks with a dense network of microcracks. Despite this, the fibers are unable to prevent crack initiation at the nanoscale. Concrete must be engineered at the nanoscale, where its chemical and mechanical properties can be truly enhanced, according to the research community. To be suitable for future emerging structural applications, these materials must have improved durability and mechanical performance, as well as functionalities that satisfy multiple uses (Dimov, 2018).
Engineered nanomaterials are classified into three categories: 0D nanoparticles, 1D nanofibers, and 2D nanosheets. Many new studies on nanomaterials such as Nano silica, Nano titanium oxide, Nano iron oxide, carbon nanotubes (CNTs), graphene oxide (GO), and graphene sheets have recently been published (Chuah et al, 2014).
A new graphene-reinforced concrete has incredible properties.

Graphene is a one-atom-thick honeycomb of carbon atoms and has world-beating mechanical, thermal, optical, and electrical properties. Flexible electronics, energy storage, nanocomposites, sensors, liquid filtration, and thermal management are just a few of the applications.
In a recent paper, researchers from the University of Exeter described a new technique for incorporating graphene into traditional concrete production using nanoengineering technology. The addition of water-stabilized graphene dispersions produces graphene-reinforced concrete with a high yield, low cost, and compatibility with the large-scale manufacturing required for use in practical applications.
They demonstrated a remarkable increase in compressive strength of up to 146 %, a 79.5 % increase in flexural strength, and a 78 % reduction in maximum displacement due to compressive loading. They also discovered improved electrical and thermal performance, including an 88 percent increase in heat capacity. Furthermore, they demonstrated a nearly 400% reduction in water permeability when compared to standard concrete. This is a highly desired property for long-term concrete structure durability, and it makes this novel composite material ideal for construction in flood-prone areas.
Finally, the researchers demonstrated that incorporating graphene into currently used concrete would result in a 50% reduction in the amount of concrete required while still meeting building loading specifications. This would result in a 446 kg per ton reduction in carbon emissions from cement manufacturing (Dimov, 2018).
The smart concrete era has arrived.
Because dry concrete does not effectively conduct electricity, adding the function of high electrical conductivity has been a long-term goal of material scientists. Large amounts of magnetite, steel fibres, and synthetic and natural forms of carbon, including graphite, were used in previous attempts, but they did not achieve high levels of performance. Furthermore, the high active material loadings required had a negative impact on cost, strength, corrosion and abrasion resistance, maintenance costs, and weight (Meyer et al, 2018).
At the Mobile World Congress 2019 in Barcelona, the cement manufacturer Italcementi, which is part of the international Heidelberg Cement Group and a member of the Graphene Flagship Consortium, displayed a mock-up that shows the many possibilities of a structural slab with a thin layer of a proprietary graphene-cementitious composite.
Italcementi researchers have modified the typical insulating behavior of cementitious compounds by incorporating graphene into concrete, allowing electric current to pass through. Underfloor heating could benefit from the electrically conductive ‘graphene concrete,' which could provide a long-term, low-maintenance alternative to plumbed hot water installations.
Furthermore, as a solid-state heater, the technology may enable more rapid and environmentally friendly ways of clearing ice and snow from houses, major transportation routes, and airports without the use of corrosive salt and antifreeze chemicals, thereby ensuring people's safety, material durability, and environmental impact.
Sensors are being installed everywhere as part of the Internet of Things (IoT) trend. Because of their widespread use, a new graphene-based smart concrete can create sensors across an entire surface; a whole building, road, or piece of machinery, rather than just a specific area within a structure or an add-on device, can be a sensor.
The use of graphene to create smart sensors that report real-time stress, temperature, and moisture changes could be the next big green building breakthrough. Conductivity enables ‘self-sensing' solutions, which can monitor stresses, deformations, and cracking states of concrete in real time without the need for additional sensors or their effective integration.
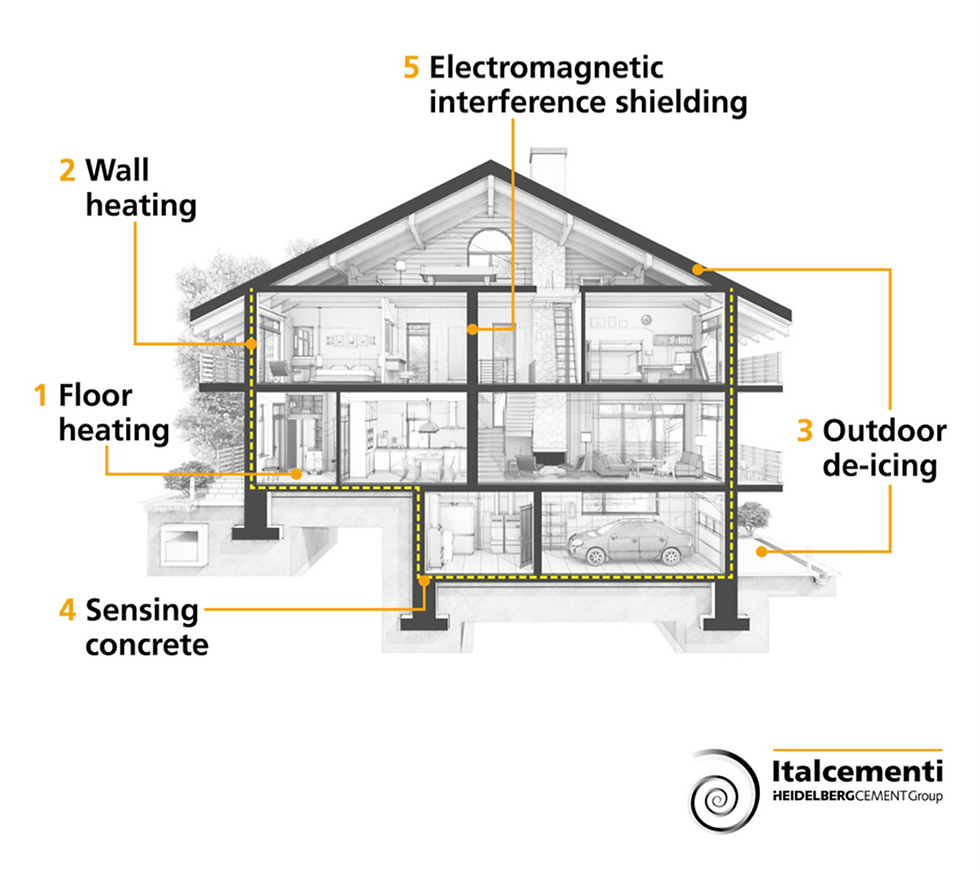
A diagram depicting the potential applications of graphene concrete in the future home. (Photo courtesy of Italcementi's public relations office)
Strain is an important indicator of structural response to external loads and for identifying damage among the wide variety of monitoring parameters (e.g., acceleration, temperature, pH, and pressure). Localized changes in structural strains, for example, may indicate the presence of cracks or impact damage. These are solutions that will allow for timely intervention at the onset of early signs of structural and infrastructure degradation, with significant benefits in terms of structure durability (Wang et al, 2017).
Anti-static flooring and EMI shielding (radio frequency interference) in buildings, as well as cost-effective grounding and lightning strike protection for a variety of infrastructure from bridges to wind turbines, are all current applications.
Italcementi has also been leading an international project to further develop graphene-based photocatalytic cement depollution. The project's goal is to improve the catalytic principle's effectiveness and extend its sensitivity in low-light situations.
Because of the increased range of heating, sensing, and other conductive concrete functions, electrically conductive concrete is already bringing great innovations in mobility by enabling inductive (wireless) charging technologies for electric vehicles under dynamic (driving) as well as stationary (parking) conditions.
Real-world applications' challenges
It’s possible to outline a significant commitment in the field of concrete re-engineering, to make concrete a more sustainable material.
The addition of graphene nanosheets to the concrete mixture appears to be a very versatile and promising solution in terms of raw material consumption, durability, and particularly for the development of applications for smart buildings and smart cities, among the possible solutions to the problem of concrete sustainability. Protective paints, self-cleaning applications, more efficient LED lights, more resistant and durable steel, more efficient solar panels, and so on have all shown that graphene has a lot of potential.
Graphene's adoption in the construction sector is not without challenges, despite predictions from academia and industry that it has a bright future.
The lack of confidence in graphene in the supply chain is one of the most significant challenges for its real-world application. The difficulty in finding a dependable graphene supplier could be a stumbling block. For example, existing suppliers face the challenge of measuring a statistically representative quantity of nanoscale flakes for each batch of material on a ton scale (Pollard, 2019).
Increasing the concentration of graphene in solvents and dispersions is another challenge for graphene producers who are significantly increasing their production volumes. Because graphene concentrations are typically 1g/L, transporting it is neither cost-effective nor environmentally friendly (Weis and Charpentier, 2019).
To avoid repeating past mistakes with new substances such as the coolant PCB, the insecticide DDT, the biocide TBT, benzene, halogenated hydrocarbons, and others, it is critical to advance knowledge about the impact graphene can have on the environment and health. The emissions from graphene for all of its possible relevant applications, the toxicological aspects, persistence in environmental media, hydrophobicity, and the evolution of the physical form of nanographene sheets are all factors to consider when developing comprehensive risk assessments (Arvidsson, 2013).
Extensive research has recently been conducted to correlate the physicochemical characteristics of graphene and related materials to biological effects, with a particular focus on the potential interactions of graphene-based materials with key target organs as well as bacteria, algae, plants, invertebrates, and vertebrates in various ecosystems.
These studies revealed that a classification based on lateral dimensions, number of layers, and carbon-to-oxygen ratio allowed for the description of the parameters that affect graphene's toxicology. These considerations can help guide future material development and applications (Fadeel et al, 2018).
More research is likely needed to fully exploit the potential of the so-called wonder material without endangering the environment or human health, but graphene or its variants will most likely become a part of our cities and homes in the coming years, bringing significant benefits to daily life.
References:
A. Favier, C. D. Wolf, K. Scriveren and G. Habert, “A sustainable future for the european cement and concrete industry; Technology assessment for full decarbonisation of the industry by 2050,” ETH Zurich, EPFL, May 2018.
A. Pollard, “When will we get graphene in civil engineering?,” January 2018. (Online). ICE, Institution of Civil Engineers, Accessed April 30, 2019.
B. Fadeel, C. Bussy, S. Merino, E. Vázquez, E. Flahaut, F. Mouchet, L. Evariste, L. Gauthier, A. J. Koivisto, U. Vogel, C. Martín, L. G. Delogu, T. B. Thurnherr, P. Wick, D. B.S. Pierre, R. Hischier, M. Pelin, F. C. Carniel, M. Tretiach, F. Cesca, F. Benfenati, D. Scaini, L. Ballerini, K. Kostarelos, M. Prato, A. Bianco, “Safety Assessment of Graphene-Based Materials: Focus on Human Health and the Environment,” ACS Nano, Vol.12, pp. 10582-10620, Nov. 2018.
D. Dimov, I. Amit, O. Gorrie, M. D. Barnes, N. J. Townsend, A. I. S. N. F. Withers, S. Russo and M. F. Craciun, “Ultra high performance nanoengineered graphene–concrete composites for multifunctional applications,” Advanced Functional Materials, Vol. 28, April 2018.
J. E. Weis and S. Charpentier, “Graphene Research and Advances, Report June 2018,” May 2018, SIO Grafen, Graphene Research and Advances.
J. Meyer, S. Beardsmore, J. Mills and S. Raevskiy, “Integrated advanced materials unlocking the future of graphene,” Talga Resources, Non-Independent Research, Nov. 2018.
L. Wang, K. Loh, R. Mousacohen and W.H. Chiang, “Printed Graphene-Based Strain Sensors for Structural Health Monitoring,” Conference: ASME 2017 Conference on Smart Materials, Adaptive Structures and Intelligent Systems, Snowbird, Utah, USA, September 18-20, 2017, ASME.
R. Arvidsson, S. Molander, B. A. Sandén, “Review of Potential Environmental and Health Risks of the Nanomaterial Graphene,” Human and Ecological Risk Assessment, Vol. 19, pp. 873-887, May 2013.
S. Chuah, Z. Pan, J. G. Sanjayan, C. M. Wang and W. H. Duana, “Nano reinforced cement and concrete composites and new perspective from graphene oxide,” Construction and Building Materials, Vol 73, pp. 113-124, Dec. 2014.
Comments